Improving quantum error correction circuits by 70% with quantum control
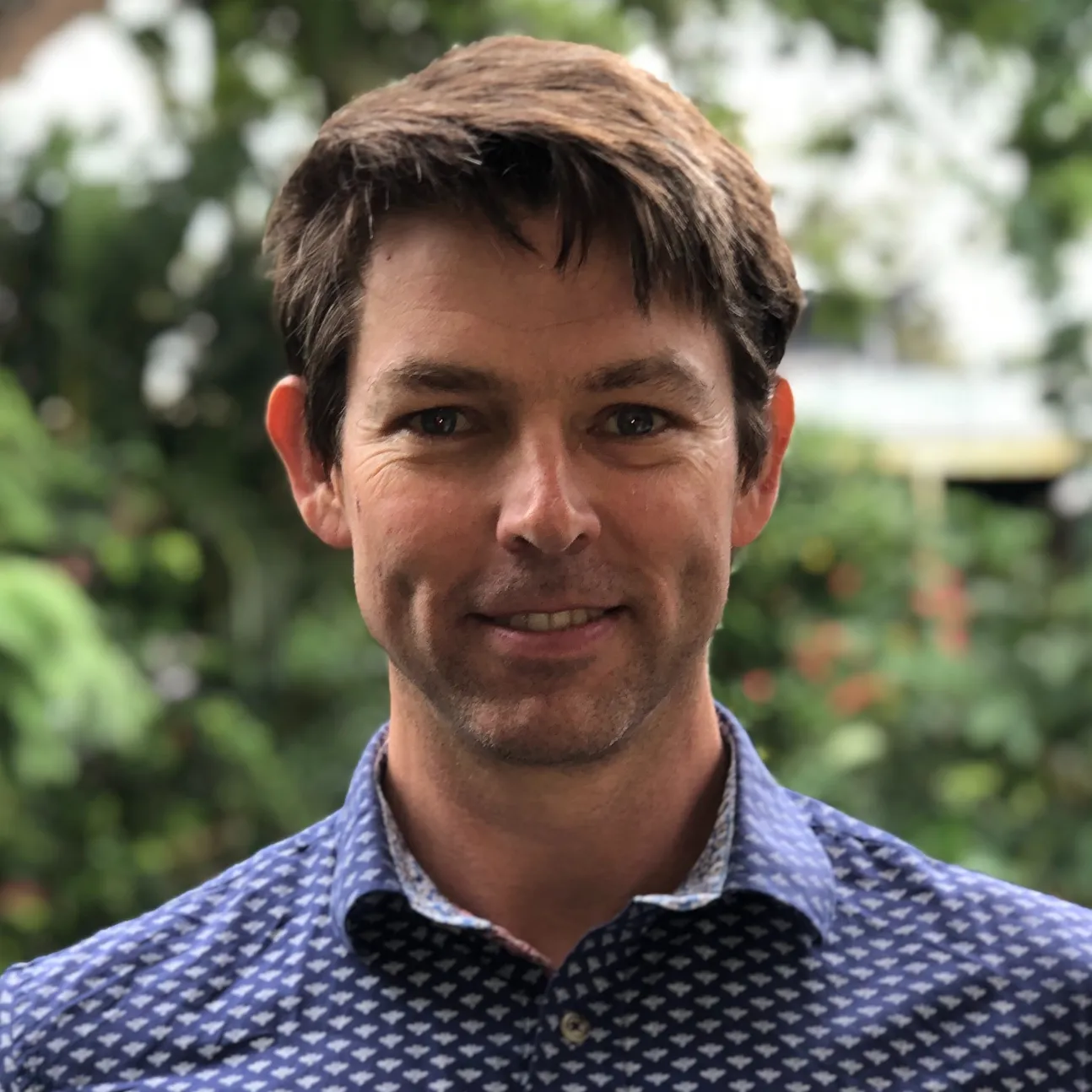

Ushering in an era of large-scale quantum computers with quantum error correction made possible by the error suppression technologies within Fire Opal.
- Problem: Quantum error correction (QEC) is fundamental to large-scale quantum computing, but currently hurts more than it helps because hardware errors are so prevalent.
- Solution: Combining QEC encoding with the deterministic error suppression techniques delivered by Fire Opal software reduces the impact of algorithmic errors so that quantum error correction can become more useful.
- Outcome: Combining a five-qubit QEC code with Fire Opal improved the code’s ability to correctly identify errors by 70% on real commercial quantum computers.
One of the fundamental challenges facing quantum technology is dealing with noise and errors. Quantum bits (qubits) are noisy, which results in the corruption of their quantum state. Dealing with these errors in order to build reliable quantum computers is the biggest challenge facing their development today.
Prevention is better than cure
Quantum hardware makers are working to operate at the limits of their hardware - our focus is to help get the greatest possible performance from today’s systems.
In a recent breakthrough, we demonstrated the utility of quantum control for reducing the severity of errors - ultimately improving the performance of quantum algorithms by up to 9,000x on real hardware. This work demonstrates the power of our Fire Opal technology to suppress errors deterministically at the hardware and circuit levels.

In order to reach large-scale quantum computation, hardware developers will eventually need to incorporate full “quantum error correction” into their platforms.
QEC enables the residual errors that creep in to be identified and corrected using a special algorithm. Even with unreliable components, QEC permits, in principle, the realization of arbitrarily large quantum computers - and many applications need really large quantum computers!
QEC is recognized as being essential to the long-term future of quantum computing. And as it turns out, Q-CTRL’s error suppression technology is essential to the viability of QEC.
“Say again, over.”
The idea of error correction is easily understood in a classical setting with the “repetition code” in which 1 classical bit of logical information is encoded into several physical copies. For example, if we want to ensure that the logical bit string “0, 0, 1” is reliably transmitted and received, we might send the triply-redundant encoded version: “000, 000, 111” by repeating each element multiple times.
If one of the redundantly encoded bits changes from 0 → 1 so that, for example, the bit string “001, 000, 111” is received, the error can be identified by a “majority vote” within each block of three bits to recover the original message.
More sophisticated codes are used in practice - such as in mobile phone or other radio transmission - but the basic idea of redundant encoding allows reliable communication over a noisy channel. These codes all share the idea of an “error syndrome”: some additional data is extracted from the encoded information, helping to infer what errors were likely, and therefore how to correct them. Just like in medicine, the syndrome is used to diagnose an underlying problem and to propose a cure.
QEC uses the principles of redundancy to provide syndrome data to reliably correct quantum information. However, there are two challenges presented by quantum physics:
- It is impossible to copy (“clone”) arbitrary quantum states, and;
- Measuring a quantum state intrinsically changes it.
Fortunately, it is possible to circumvent these hurdles with special tricks that allow for information encoding and partial measurements; the theory of quantum error correction is now well established and has seen many foundational demonstrations in the community.
Quantum error correction is a quantum algorithm - let's fire it up!
So, why isn’t QEC widely used already? It has not yet reached a point where it delivers actual net benefits to the user. This is due to all the overhead required in the process of encoding and identifying errors through syndrome measurements. In current demonstrations of QEC, on balance, more errors are introduced than are corrected.
If we take a step back, we can actually find a new path forward.
First, we have to see that the basic elements required to implement a QEC code are the same as any other quantum algorithm - quantum gates arranged into circuits and measurements that provide the error syndrome. This output information is then used to correct the error, in a larger control loop, which is, in principle, similar to those used in variational algorithms for quantum chemistry or finance.
This point warrants emphasis - while QEC is fundamental mathematically to quantum computer science, it is in practice closely related to other algorithms run on quantum computers. This reveals a new path to augment QEC protocols with techniques already validated to improve quantum algorithms run on near-term devices.
At Q-CTRL, we look at the problem of error in quantum computers holistically, from the lowest levels of hardware stabilization through to efficient execution of QEC protocols. To this end, we recently combined Fire Opal - which systematically enhances the hardware performance of any algorithm - with the encoding and error-syndrome measurement protocol from QEC in order to improve the overall performance of QEC executed on superconducting qubits.
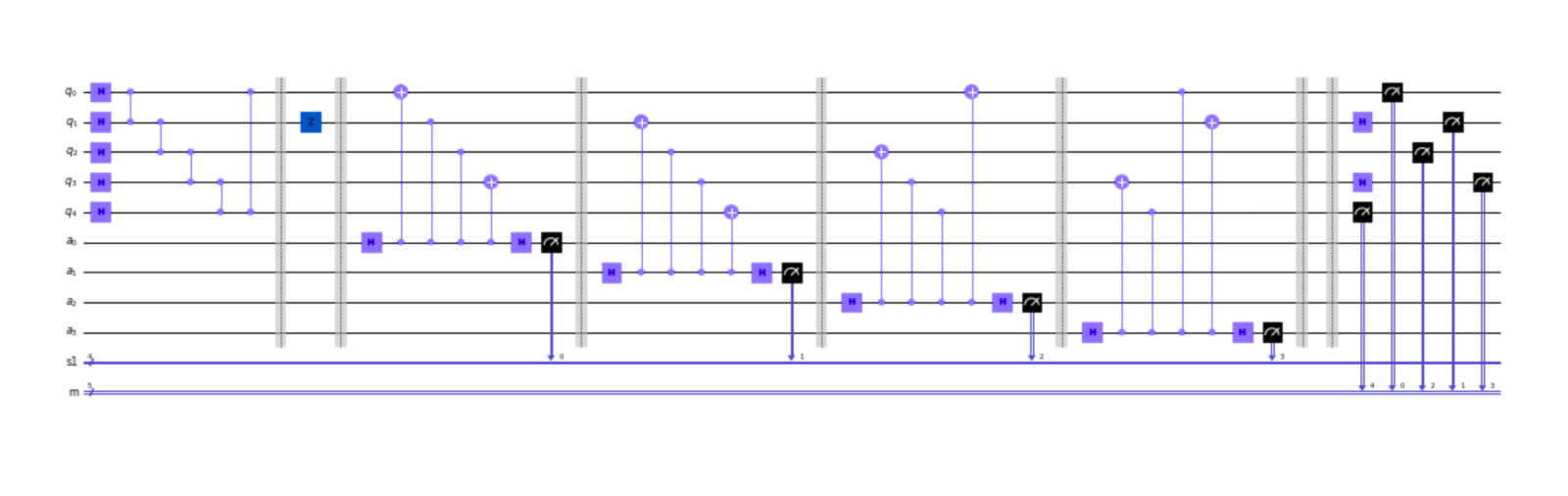
“We implemented the smallest quantum error correcting code, called the 5-qubit code, on IBM’s quantum computing hardware,” said Dr Yuval Baum, Lead Scientist, Quantum Control. “This required a total of 9 qubits, 5 of which were the data qubits, and the others were helper qubits. To measure the performance enhancement, we injected artificial errors with well-known syndromes into the data qubits, and compared that with the measured syndrome.”
The team found an improvement of up to 70% in the accuracy of the syndrome extraction on production hardware.
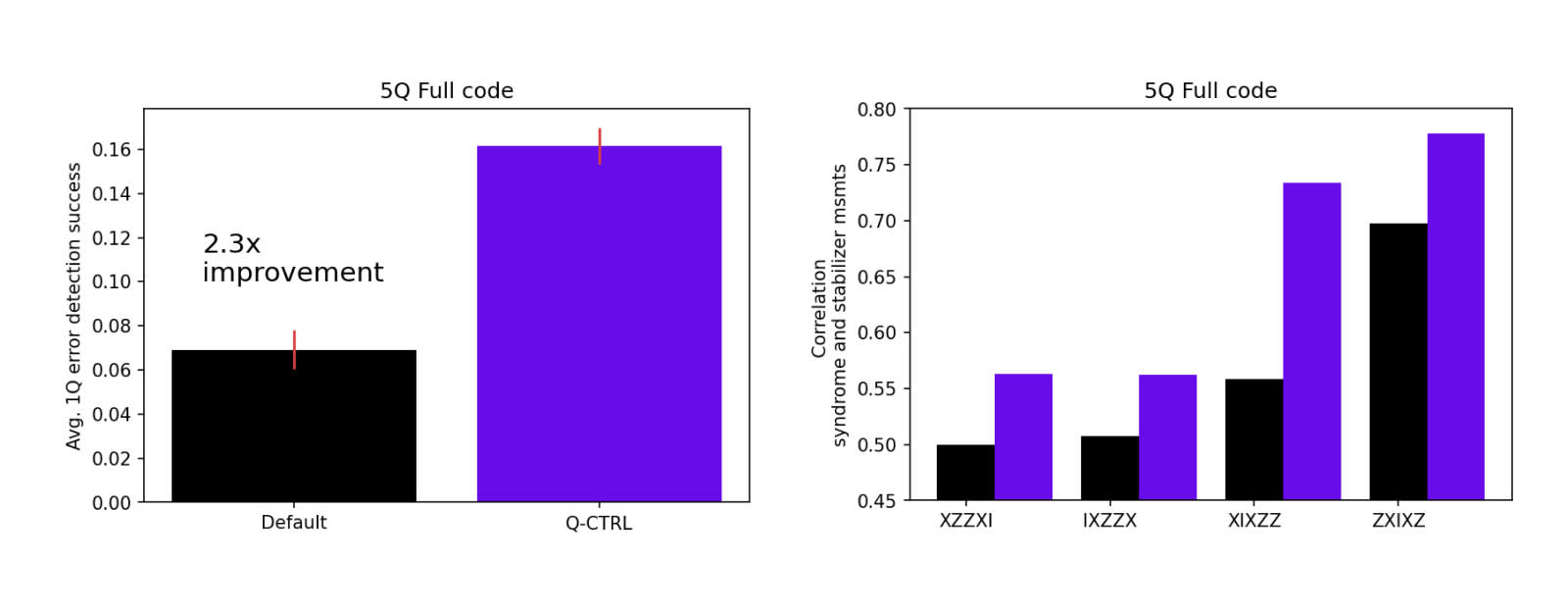
“Our tools will enable hardware makers to reach the ultimate performance limits of their devices and suit any quantum error architecture that they have chosen,” said Dr Pranav Mundada, Senior Scientist, Quantum Integration. “This demonstration is just the start, and we look forward to working with our partners to help them beat the quantum error correction break-even point on their platforms.”
Quantum control is an exceptionally powerful tool to deliver performance enhancement in quantum computers, from the bottom of the stack to the top. These new results demonstrate again the power of Fire Opal in improving the performance of any algorithm - even QEC.
Extracting every iota of performance from available hardware will be essential in transforming quantum error correction into a beneficial tool as quantum computing hardware matures. Fire Opal and its world-leading capabilities in deterministic error suppression are critical to making quantum computers useful, now and in the long term.